Sequencing Technologies and Methods Explained
- 9th February 2024
- Posted by: Breige McBride
- Categories: Gene Expression Analysis, Sequencing, Single Cell Analysis
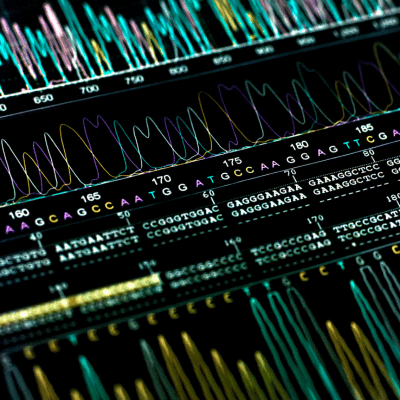
When it comes to genomic sequencing, it can feel like there are endless sequencing technologies and sequencing methods. Although there are just three key types of sequencing technologies, new sequencing methods are continually being developed. The ever-growing list of sequencing methods is what can sometimes confuse people. To make thing clearer, this blog will provide:
- An overview of the 3 main sequencing technologies
- An explanation of the 2 main categories that sequencing methods fall into (DNA sequencing and RNA sequencing)
- A list of DNA sequencing methods (periodically updated)
- A list of RNA sequencing methods (periodically updated)
However, before we get to explaining the different genomic sequencing technologies and methods, it is important to have a basic understanding of what genomic sequencing is:
What is genomic sequencing?
To understand what genomics sequencing is, you first need to understand what genomics is. Genomics is the study of genomes and a genome is an organism’s complete set of DNA. (DNA is comprised of four bases, the nucleotides Adenine, Thymine, Cytosine and Guanine, which are represented by the letters A, T, C & G. These bases pair together in base pairs. A is always paired with T and G is always paired with C). Genomic sequencing is a technique for ‘reading’ DNA. In other words, genomic sequencing allows us to work out the order of all the As, Ts, Cs and Gs in an organism’s DNA. (Some genomic sequencing technologies can also read RNA, comprised of As, Us, Cs and Gs.)
By allowing us to read DNA and RNA, genomic sequencing opens up many analyses to further our understanding of health and disease.
Since genomes contain so many letters (a human genome contains around 3 billion base pairs) it isn’t yet possible work out the order of all the letters in one go. Sequencing machines typically ‘read’ short sections of DNA, around 150 letters at a time. This is known as “short-read sequencing”. However, some sequencing machines can read longer sections of DNA, providing “long-read sequencing”.
Genomic Sequencing Technologies
The evolution of DNA sequencing technologies has been marked by significant milestones, each contributing to increased speed, accuracy, and cost-effectiveness. You can learn about these below.
Sanger Sequencing (1st generation sequencing technology)
The first generation of sequencing technology was Sanger sequencing. Although it was developed by Frederick Sanger in 1975, it is still widely used today. It works via the selective incorporation of chain-terminating dideoxynucleotides during DNA synthesis, producing fragments of varying lengths that can be separated by gel electrophoresis.
Sanger sequencing is accurate and reliable for determining the nucleotide sequence of short DNA fragments. It is also possible to apply Sanger sequencing to longer DNA fragments, up to 1000 bp. However, Sanger sequencing is most effective with short DNA fragments as it becomes less practical and cost-effective to use as the length of the DNA fragment increases.
Since it reliably produces accurate results, Sanger sequencing is a trusted choice in molecular biology and genetics research. Researchers often use it for sequencing individual genes and confirming the identity of cloned DNA fragments. It is also the gold standard method for detecting single nucleotide variants. However, it is limited by its labour-intensive nature and the analysis of relatively short DNA fragments.
Next Generation Sequencing (2nd generation sequencing)
The advent of high-throughput sequencing technologies in the 21st century marked a paradigm shift in genomic research, with the introduction of next-generation sequencing platforms. These technologies revolutionized genomics by enabling the sequencing of entire genomes, transcriptomes, and metagenomes in a relatively short time.
Next Generation Sequencing (NGS) refers to a group of short-read “massively parallel” sequencing technologies and long-read sequencing technologies developed after Sanger sequencing. These allowed for massively parallel sequencing of DNA fragments, significantly increasing throughput and reducing costs. However, referring to them all under the term NGS is confusing, as they work in very different ways. Also, since long-read sequencing was developed after short-read sequencing, it can be considered as “third generation sequencing”.
Short-read massively parallel sequencing
These are high-throughput sequencing technologies which allow for the simultaneous sequencing of millions of DNA or RNA fragments. However, each fragment is sequenced independently. After this, the sequences are computationally reconstructed to generate the complete genomic information. Since these technologies can sequence millions of DNA fragments simultaneously, they are faster and more cost-effective than Sanger sequencing. There are various clinical applications of short-read sequencing, such as Whole Genome Sequencing (WGS), Whole Exome Sequencing (WES), RNA sequencing (RNA-seq) and more.
Examples of short-read massively parallel sequencing technologies include:
-
-
- Sequencing by synthesis platforms from Illumina
- Semiconductor sequencing (Ion Torrent) platforms from Thermo Fisher Scientific
- Sequencing by Oligonucleotide Ligation and Detection (SOLiD) platforms from Thermo Fisher Scientific
-
Long-read sequencing (3rd generation sequencing)
As demand for longer read lengths and improved accuracy grew, long-read sequencing emerged. Long-read sequencing technologies can sequence long strands of DNA or RNA, without needing to split these into smaller fragments. This enables the sequencing of longer stretches of DNA or RNA than short-read sequencing. Like short-reading sequencing, long-read sequencing also has clinical applications in WGS and WES, as well as many others. However, since long-read sequencing reads larger chunks of DNA at once, it is better than short-read sequencing for reading long repeated sections and large-scale structural variations in DNA.
Examples of long-read sequencing technologies include:
-
-
-
- Single Molecule Real-Time (SMRT) sequencing from Pacific Biosciences (PacBio)
This technology utilises circular templates and real-time observation of DNA polymerase activity to generate longer reads. It addresses some of the limitations of short-read technologies, providing insights into complex genomic regions, structural variations, and epigenetic modifications.
- Single Molecule Real-Time (SMRT) sequencing from Pacific Biosciences (PacBio)
- Nanopore sequencing. Companies that provide nanopore sequencing technologies include Oxford Nanopore Technologies, Bionano Genomics, Illumina and Pacific Biosciences.
Nanopore sequencing involves passing a DNA or RNA strand through a nanopore, and changes in electrical current are used to identify the nucleotides. Nanopore sequencing offers the advantages of long reads and real-time analysis, making it suitable for applications such as de novo genome assembly, structural variant detection, and direct RNA sequencing.
-
-
Sequencing Methods
Now that you have an understanding of sequencing technologies, it is time to explain sequencing methods. Sequencing methods are the methodologies or approaches which determine the order of nucleotides in a DNA or RNA molecule. On the other hand, sequencing technologies (such as those above) are the machines or platforms that implement these methods.
Since both DNA and RNA can be sequenced, DNA sequencing and RNA sequencing are the two main categories that sequencing methods belong to. There are many DNA and RNA sequencing methods as these are continually being developed. However, some of the key methods are detailed below:
DNA sequencing methods
Assay for Transposase-Accessible Chromatin sequencing (ATAC-seq)
ATAC-seq is an DNA sequencing method for assessing chromatin accessibility on a genome-wide scale. It does this by identifying DNA regions that are accessible for transcription factor binding. The ATAC-seq workflow involves transposition, DNA purification, amplification, sequencing and analysis. Applications that ATAC-seq can help with include studying chromatin dynamics and understanding gene expression regulation.
If you need help with ATAC-seq data analysis, contact Fios Genomics for a quote.
Chromatin Immunoprecipitation Sequencing (ChIP-seq)
ChIP-seq is a method for studying the protein-DNA interactions within a cell. It combines Chromatin immunoprecipitation assays with DNA sequencing in order to interrogate the physical binding interactions between protein and DNA. Researchers use it to study protein-DNA interactions such as histone modifications and transcriptome factor binding. You can learn more about how ChIP-seq works here.
If you need help with ChIP-seq data analysis, contact Fios Genomics for a quote.
Metagenomic Sequencing
Metagenomic sequencing involves the analysis of genetic material recovered directly from samples containing microbial communities. It enables the study of microbial diversity in various environments, such as the human microbiome, and environments such as soil.
If you need help with metagenomic sequencing data analysis, request a quote.
Methylation Sequencing (methyl-seq)
The purpose of methylation sequencing is to identify and analyse the DNA methylation patterns in a genome. The methyl-seq process involves bisulphite conversion, DNA Fragmentation, PCR amplification, sequencing and analysis. You can learn more about how this process works here. Methylation sequencing helps researchers to understand the epigenetic modifications involved in gene expression, development and disease.
If you need help with methyl-seq data analysis, contact Fios Genomics for a quote.
Targeted Amplicon Sequencing
Targeted amplicon sequencing involves amplifying and sequencing specific genomic regions of interest. It is employed in microbiology for identifying and characterizing microbial pathogens, aiding in disease diagnosis and monitoring outbreaks, while in the field of pharmacogenomics, targeted sequencing is used to identify genetic variants that influence an individual’s response to drugs, facilitating personalized medicine approaches.
If you need help with targeted amplicon sequencing data analysis, contact Fios Genomics for a quote.
Whole Exome Sequencing (WES)
WES focuses on sequencing the protein-coding regions of the genome, known as exons. While exons represent a small fraction of the entire genome, they harbour a significant proportion of disease-associated variants. WES is widely used in clinical genetics to identify mutations responsible for rare genetic disorders, cancer, and other hereditary conditions. It also plays a crucial role in identifying somatic mutations in cancer genomes, aiding in the understanding of tumour evolution and informing personalized treatment strategies.
Whole Genome Sequencing (WGS)
WGS determines the complete DNA sequence of an organism’s entire genome. By reading the nucleotide bases of an organism’s DNA and determining their order, WGS provides a comprehensive view of an organism’s genetic material. This enables researchers to identify variations, mutations, and genetic markers within an individual’s or a species’ genome. WGS is useful for applications such as; diagnosing rare genetic disorders, developing personalised medicines and understanding genetic variations within populations. By facilitating population-wide studies, WES helps researchers understand genetic diversity, migration patterns, and population history.
If you need help with WGS data analysis, contact Fios Genomics for a quote.
These applications represent just a fraction of the diverse uses of DNA sequencing technologies, emphasizing their impact on fields ranging from medicine to ecology. Continual advancements in sequencing methodologies further enhance the capacity to extract meaningful insights from the vast and intricate landscape of genomic information.
RNA sequencing (RNA-seq) methods
Alternative Splicing and Isoform Analysis
RNA sequencing enables the study of alternative splicing, a process where a single gene can produce multiple mRNA isoforms by selectively including or excluding certain exons. Researchers can use RNA sequencing to identify and quantify alternative splicing events, providing a nuanced understanding of transcript diversity and functional implications. Investigating alternative splicing patterns in diseases such as cancer can reveal aberrant isoforms associated with tumorigenesis, potentially serving as diagnostic or prognostic markers.
If you need help investigating alternative splicing, contact Fios Genomics for a quote!
Bulk RNA Barcoding and Sequencing (BRB-seq)
BRB-seq is a gene expression analysis method which uses early multiplexing to produce 3′ cDNA libraries for multiple samples. This means that for analysing gene expression across multiple samples it is much quicker and more cost-effective than some traditional RNA sequencing methods. You can learn more about BRB-seq and how it works here.
Looking for BRB-seq data analysis? Request a quote now!
Direct RNA sequencing
While conventional RNA sequencing methods usually involve converting RNA into complementary DNA (cDNA) before sequencing, with Direct RNA sequencing the RNA molecules are sequenced without being converted into cDNA. This eliminates the potential biases and artifacts that can be introduced when RNA is converted to cDNA.
If you need help with Direct RNA sequencing data analysis, contact Fios Genomics for a quote.
Digital RNA with pertUrbation of Genes (DRUG-seq)
DRUG-seq is an RNA sequencing method that helps researchers understand how genes respond to drug treatments. By integrating RNA sequencing with genetic perturbations induced by drug exposure, DRUG-seq provides a digital readout of changes in gene expression in response to specific pharmaceutical interventions. This method is therefore invaluable for deciphering the transcriptomic consequences of drug-induced perturbations, as it sheds light on molecular mechanisms, helps identify potential drug targets, and enhances our understanding of drug responses at the genomic level.
If you need DRUG-seq data analysis, then contact us now for a quote!
Gene Expression Profiling
RNA sequencing provides a comprehensive snapshot of the transcriptome, allowing researchers to quantify the abundance of RNA molecules and understand how genes are expressed under different conditions. By comparing RNA expression levels between different samples (e.g., healthy vs. diseased tissues or treated vs. untreated cells), researchers can identify genes that are upregulated or downregulated, providing insights into disease mechanisms and treatment responses.
Researchers can employ RNA sequencing to characterize the gene expression profiles of specific cell types within heterogeneous tissues, aiding in the identification and understanding of cell-specific functions.
If you need support with gene expression profiling data analysis, then contact us for a quote!
Micro RNA sequencing (miRNA-seq)
miRNA-seq is the sequencing of micro RNAs (miRNAs) which are short, single-stranded RNA molecules. These are usually composed of between 20 and 22 nucleotides. Researchers use miRNA-seq to identify and quantify miRNAs in a biological sample, as well as to provide insights into their expression levels and potential regulatory functions.
Looking for miRNA-seq data analysis? Contact us now for a quote!
mRNA sequencing (mRNA-seq)
mRNA refers to messenger RNA, so mRNA-seq is messenger RNA sequencing. mRNA-seq is a sequencing method for analysing and quantifying the mRNA content in a biological sample. It indicates which genes are producing mRNA and provides a comprehensive assessment of the transcriptome. The mRNA-seq process involves RNA extraction, mRNA enrichment, library preparation, sequencing and analysis.
If you need help with mRNA-seq data analysis, contact Fios Genomics for a quote.
Single-cell RNA sequencing (scRNA-seq)
scRNA-seq is an RNA sequencing method that researchers use to analyse the gene expression profile of individual cells within a population. In other words, it helps them see what genes are active in each individual cell. In the context of drug discovery, scRNA-seq plays a crucial role in elucidating the heterogeneity within cell populations and understanding how individual cells respond to drug treatments.
scRNA-seq enables the identification of rare cell subpopulations, the characterization of cell-state transitions, and the exploration of molecular signatures associated with drug response or resistance. Since it provides a detailed and precise analysis of cellular responses at the single-cell resolution, scRNA-seq contributes valuable insights to drug discovery efforts. These insights then help researchers uncover novel therapeutic targets, optimize treatment strategies, and advance understanding of the complex dynamics underlying drug responses within diverse cell populations.
If you need help with scRNA-seq data analysis, contact Fios Genomics for a quote.
See also:
Single-cell vs Bulk RNA Sequencing
Bioinformatics 2024: Predictions and Challenges
NGS Platforms: Illumina vs Oxford Nanopore